Research
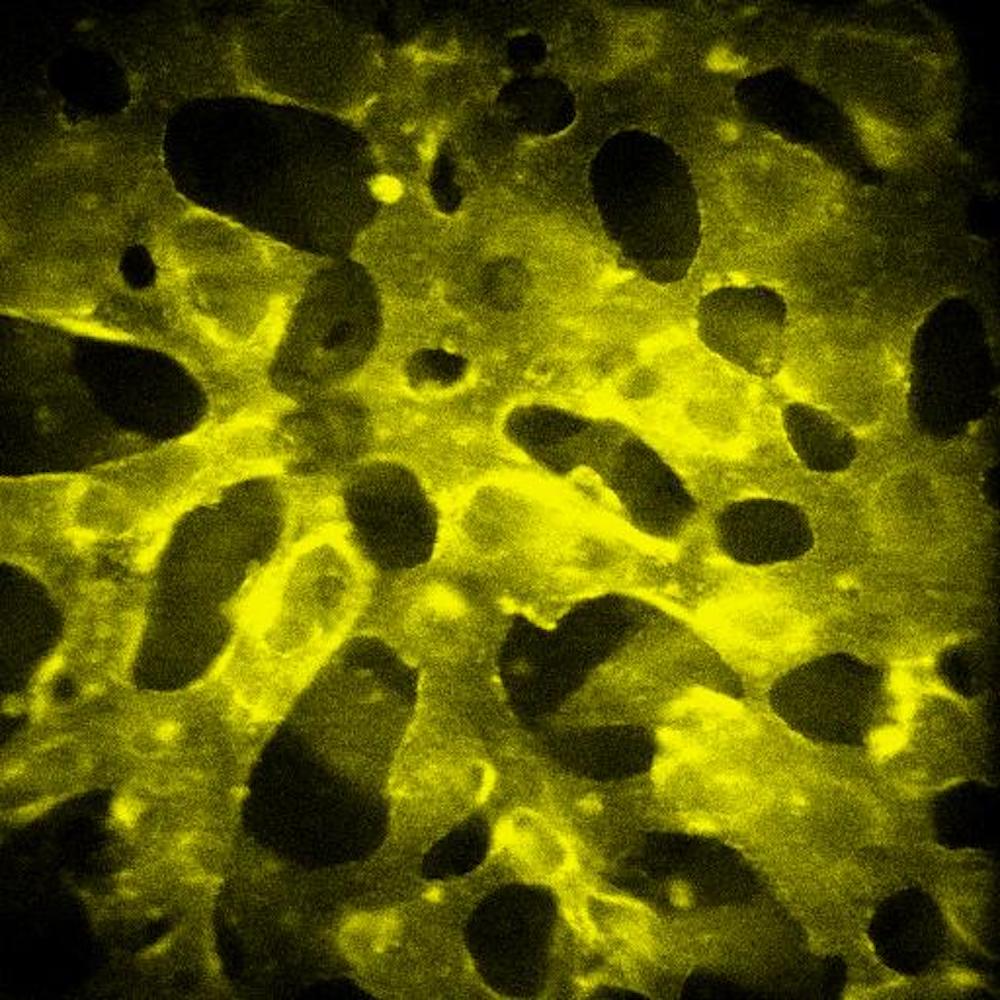
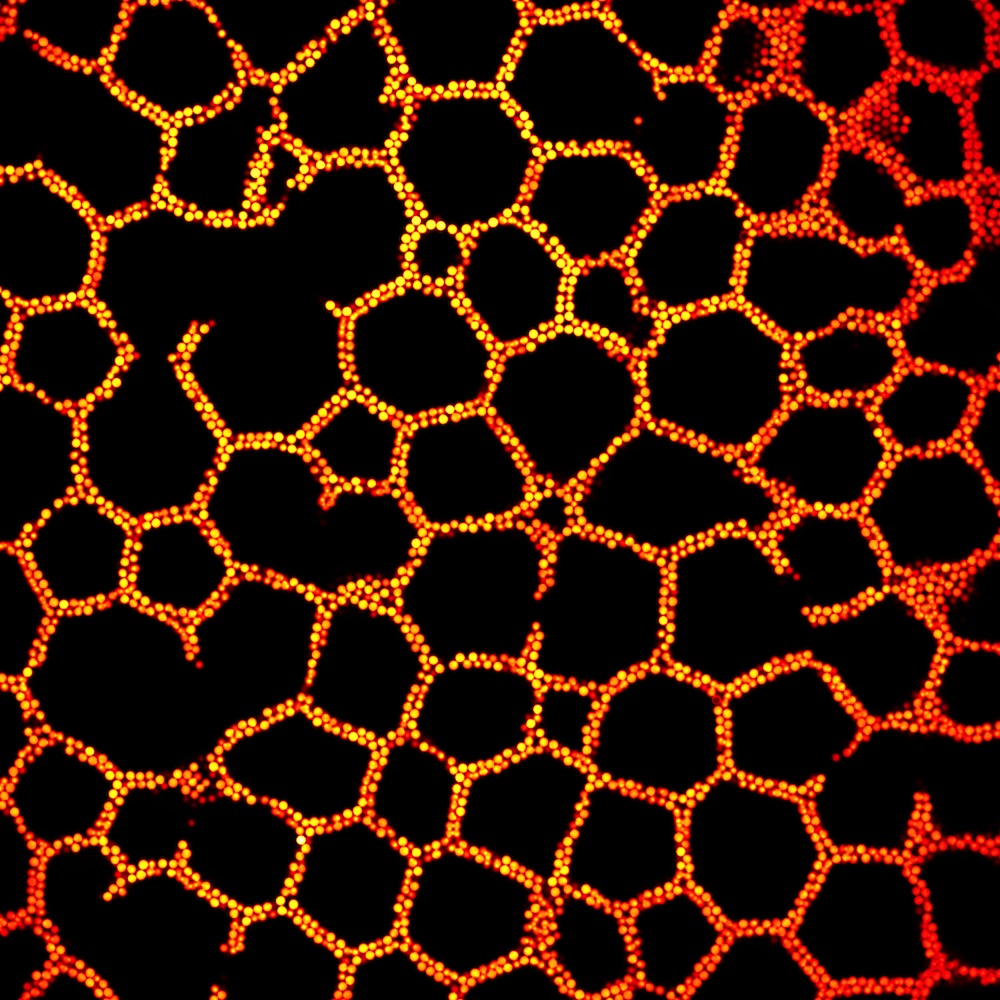
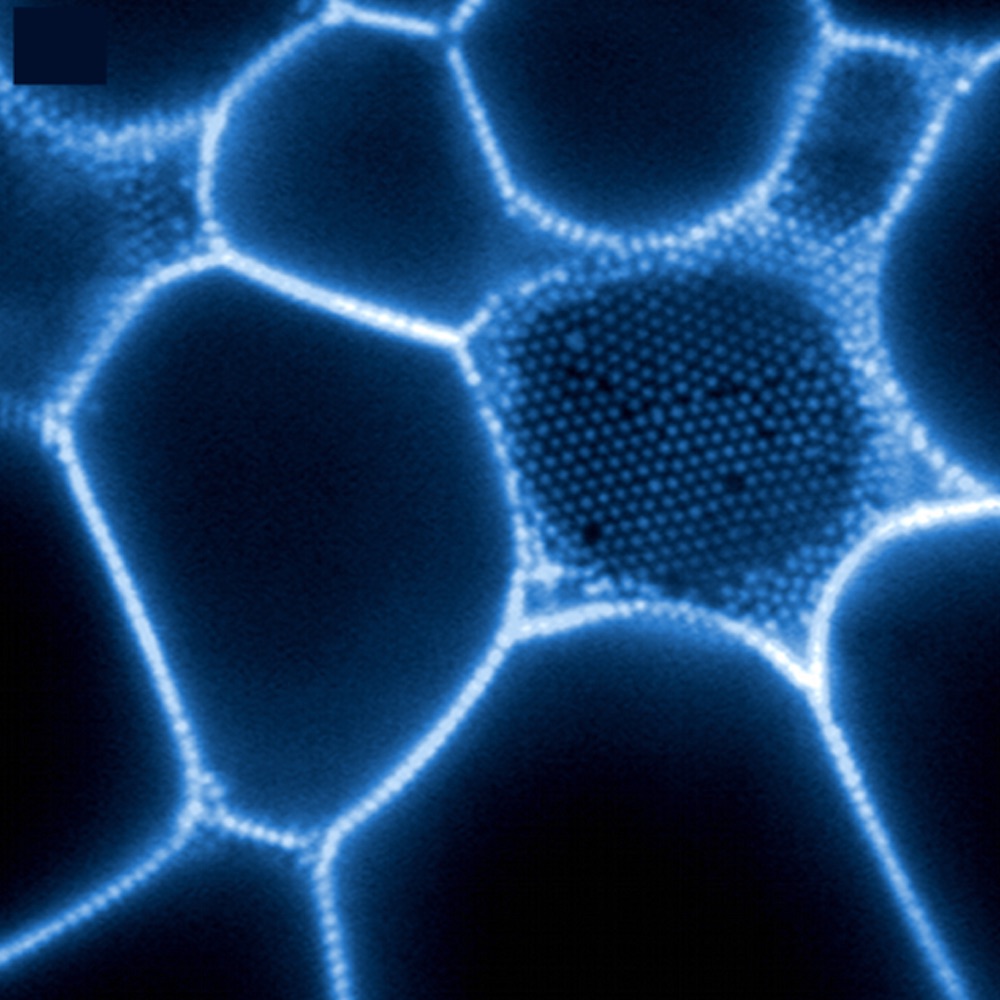
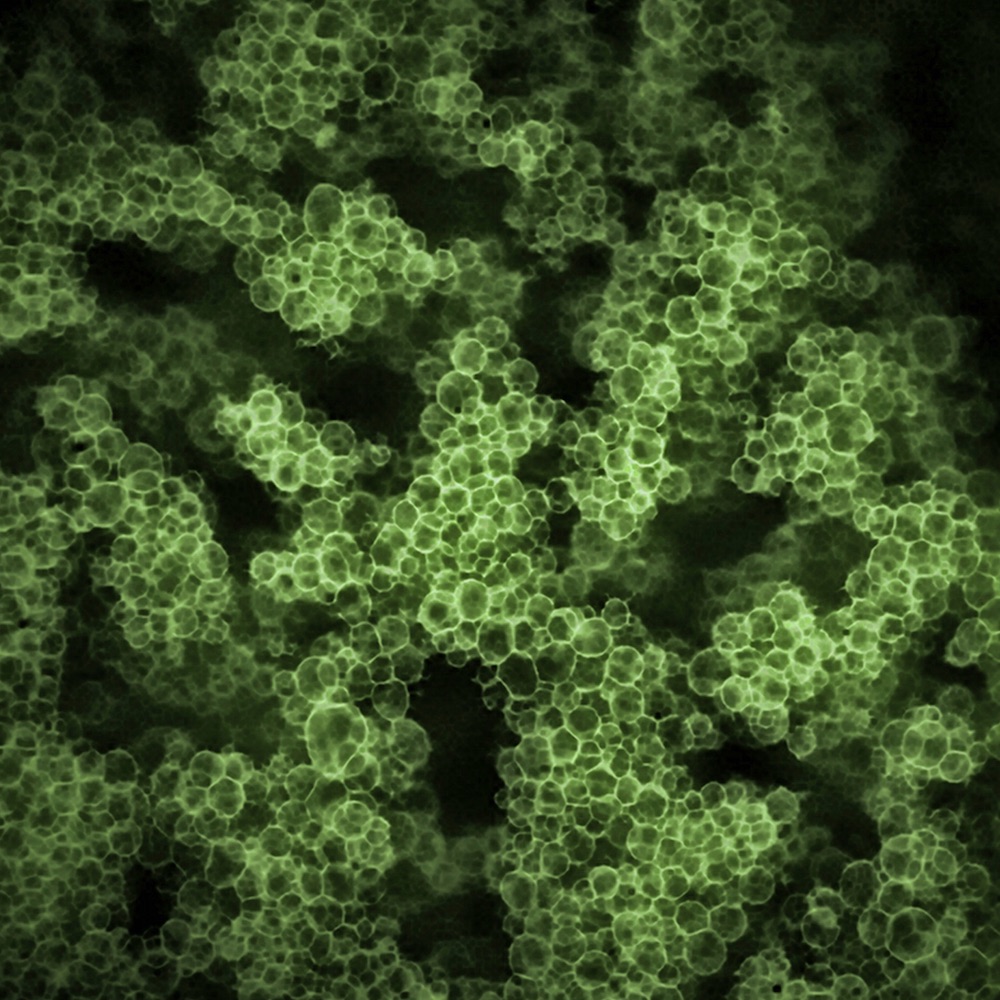
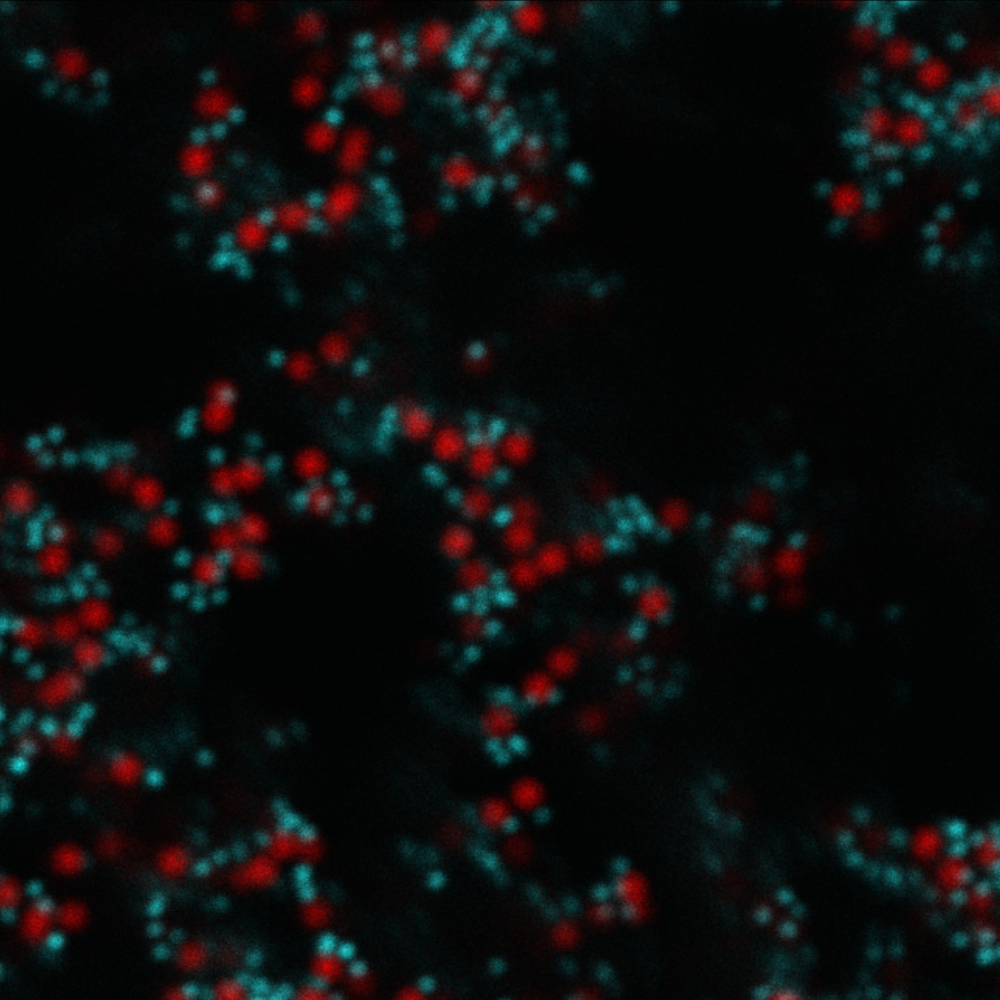
Fundamentals of Soft Matter
What makes soft materials unique and differentiates them from solids and liquids is their dynamic mechanical properties. These properties are intimately tied to the material’s microstructure, but the connection between the microstructure, dynamics, and bulk mechanical properties of many soft materials is not well understood. Our group investigates this fundamental problem, and how it can be exploited to engineer desired properties, in various classes of soft materials. Examples of past and current projects in this area are the microstructural origins of nonlinear rheology in colloidal gels, the physics of colloidal assembly at fluid interfaces, the mechanics of bicontinuous interfacially jammed emulsion gels (bijels), and the micro-dynamics of gelation and coarsening in attractive bimodal suspensions. A number of our research activities in this research thrust are performed at the International Space Station (ISS). Access to this microgravity environment allows us to recreate a simplified version of the problem at hand (without gravitational forces), as a first step in investigating and understanding its physics. We also collaborate with computational soft matter scientists, together aiming to develop better theoretical models to predict the behavior of soft materials. To learn more about the details of our projects, please contact Professor Mohraz.
Collaborators: Professor Safa Jamali (Northeastern University)
Selected references:
Impact of Particle Size on Droplet Coalescence in Solid-Stabilized High Internal Phase Emulsions. M. Kaganyuk and A. Mohraz, Langmuir, 35, 12807 (2019 (Featured on the Cover).
Microdynamics of dense colloidal suspensions and gels during constant-stress deformation. H.K. Chan and A. Mohraz, Journal of Rheology, 58, 1419 (2014)
Characteristics of Pickering Emulsion Gels formed by Droplet Bridging. M.N. Lee, H.K. Chan, and A. Mohraz, Langmuir, 28, 3085 (2012) (Featured on the Cover).